I. Introduction
II. Operating principles of SBAT
III. Applications of SBAT in biology and medicine
3.1 Cell separation using SBAT
3.2 Cell deformation using SBAT
IV. Conclusions
I. Introduction
Scientific breakthroughs often arise on the foundation of technological advancements. Notably, the progress of cell theory is intricately intertwined with the strides made in the field of microscopy. In a similar manner, recent developments in the domain of single-cell and biomolecular manipulation have played a pivotal role in propelling advancements in microbiology, molecular biology, biophysics, and bioanalytical chemistry.
One promising and emerging platform for the precise manipulation of bioparticles, spanning a wide range of sizes, is acoustic tweezers.[1,2] These innovative tools employ the interaction of sound waves with solids, liquids, and gases to exert spatial and temporal control over matter. The acoustical tweezers initially described the linear translation observed in trapped latex spheres and frog eggs within an acoustic field.[3] Since then, a considerable number of acoustic tweezer configurations have been developed to cater to diverse applications in the realms of science and engineering.[3,4,5] Many of these acoustic tweezer devices draw inspiration from their predecessor, optical tweezers.
Optical tweezers, invented in 1986, swiftly became an indispensable instrument in the fields of biology, chemistry, and physics.[6,7] They have been extensively utilized to trap various entities, including viruses, bacteria, and cells, thus enabling investigations in force spectroscopy and biomolecular manipulation. However, traditional optical tweezers necessitate intricate optical setups, encompassing high-powered lasers and high-numerical-aperture objectives, which can potentially pose risks to biological samples. To address these limitations and enhance the accessibility and versatility of particle-manipulation technology without physical contact, alternative methodologies to optical tweezers have been devised.
Acoustic tweezers present a versatile and innovative solution that overcomes prevailing limitations encountered in conventional manipulation techniques. By harnessing acoustic waves spanning the frequency spectrum from kilohertz to megahertz, acoustic tweezers enable direct and precise manipulation across an extensive length scale exceeding five orders of magnitude.[8,9] Notably, the applied acoustic power and frequencies employed in acoustic tweezers align closely with those utilized in ultrasonic imaging, a well-established and safe diagnostic modality, further enhancing their credibility and reliability.
Extensive biocompatibility studies conducted on acoustic tweezers have yielded promising results, demonstrating the ability to optimize operational parameters and mitigate potential harm to cells and small-animal models.[10,11] This inherent biocompatibility, coupled with the inherent adaptability and versatility of acoustic tweezers, positions them as a potent tool for addressing contemporary challenges in the domains of biology and biomedicine. Notably, acoustic tweezers hold substantial potential in facilitating the isolation and detection of circulating biomarkers for cancer diagnostics, thereby catalyzing advancements in early detection and precision medicine. By seamlessly integrating with existing biomedical practices, acoustic tweezers offer a promising avenue for driving scientific progress and improving patient outcomes.
Over the past few decades, there have been notable advancements in the field of Single Beam Acoustic Tweezers (SBAT), which presents a groundbreaking advancement in the field of precisely manipulating cells and organisms, offering distinct advantages over conventional techniques like standing waves.[12,13] Unlike complex setups required by standing wave techniques, SBAT use just one easily controlled acoustic beam, simplifying experiments and making it accessible across various research areas. Additionally, SBAT enables precise trapping of individual cells, ensuring gentle handling of delicate samples that could be harmed by the higher energy of standing wave systems. Recent innovations have expanded the capabilities of SBAT, enabling particle/cell separation and controlled deformation of individual cells.[14,15,16,17,18,19] These remarkable advancements measuring cellular properties have elevated SBAT to a prominent position in the field of bioparticle manipulation, attracting considerable attention from the scientific community.[20,21,22,23] This review will also present a comprehensive overview of the most recent groundbreaking achievements in SBAT, highlighting cell studies that have propelled this technology to the forefront of cell manipulation. We aim to elucidate the full potential and future prospects of SBAT in diverse scientific disciplines such as cellular biology and biophysics.
II. Operating principles of SBAT
The physical mechanism underlying SBAT bears resemblance to that of optical tweezers, as depicted in Fig. 1.[24,25] Upon incidence of a Gaussian ultrasound beam onto a single cell, the rays undergo refraction as they penetrate the cell. The rays located in proximity to the beam axis (p1) exhibit higher intensity than those situated at the beam periphery (p2). Each refraction event entails a change in the direction of the ultrasound rays, while simultaneously conserving momentum. This phenomenon engenders a gradient force that acts upon the cell.
The force arising from the directional modification of ray momentum manifests as both forward and lateral components. Symbolically, F1 and F2 represent the forces exerted on the cell by p1 and p2, respectively. The total force (Ftotal) corresponds to the vector sum of these individual forces, which aligns itself with the beam axis. Notably, due to the experimental configuration involving a petri dish, the setup exclusively encompasses transverse trapping, resulting in the displacement of the cell towards the ultrasound focus along the lateral direction (as indicated by the white arrow in Fig. 1). Moreover, when the cell precisely aligns with the ultrasound focal point, the net gradient force acting upon it becomes null, owing to the symmetrical nature of ultrasound refraction at this particular spatial location.
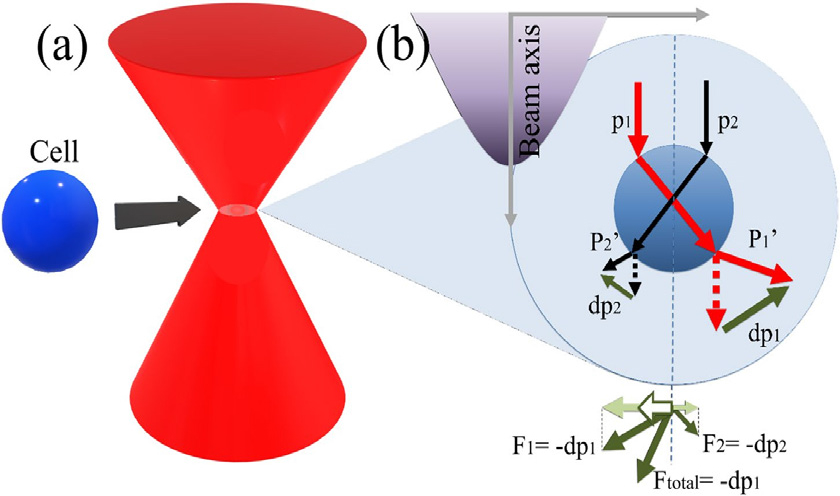
Fig. 1.
(Color available online) Mechanism of Signal Beam Acoustic Tweezers (SBAT): The mechanism of SBAT involves the manipulation of cell movement through the propagation of two rays, characterized by distinct intensities (represented by red and black arrows), within the cell. The resulting net force, represented as Ftotal, influences the direction of cell movement along the lateral axis. This directional movement is indicated by the white arrow, demonstrating the control achieved through SBAT. Fig. 1 is reprinted with permission from Reference [24], respectively, Springer Nature.
III. Applications of SBAT in biology and medicine
The remarkable versatility exhibited by SBAT positions them as a promising solution to address prevailing challenges in the fields of biology and medicine. Their capabilities span a wide range of applications, particle/cell separation and controlled deformation of single cells. As a result, SBATs are emerging as an alternative to conventional tools employed for particle and cell manipulation across diverse domains, ranging from diagnostics to the intricacies of single-cell studies.
3.1 Cell separation using SBAT
Cell separation techniques play a critical role in various biological and biomedical applications. Especially cancer cell separation techniques, designed for the isolation and characterization of cancer cells, hold significant importance in cancer research and clinical applications such as early detection and diagnosis and personalized medicine and targeted therapies. In addition, considerable research endeavors have been dedicated to quantitatively measuring the interactive forces between Red Blood Cells (RBCs) to enhance our understanding of hemodynamics and blood rheology. This is because RBC aggregation significantly impacts blood circulation and tissue perfusion, affecting various aspects such as microcirculatory blood flow dynamics and venous flow resistance. Aberrant RBC aggregation has been identified as a contributing factor in diseases associated with circulatory disorders, such as malaria and sickle cell disease.
Over the past few decades, the mechanics of cell separation technique at the cellular level have been investigated using different technologies, including Scanning Electron Microscopy (SEM), Micropipette Aspiration Technique (MAT), and Atomic Force Microscopy (AFM). Among these techniques, SBAT has gained considerable popularity due to its noninvasive nature and ability to provide cell separation (Fig. 2) and quantify the inter cellular forces (Fig. 3) at the single-cell level.
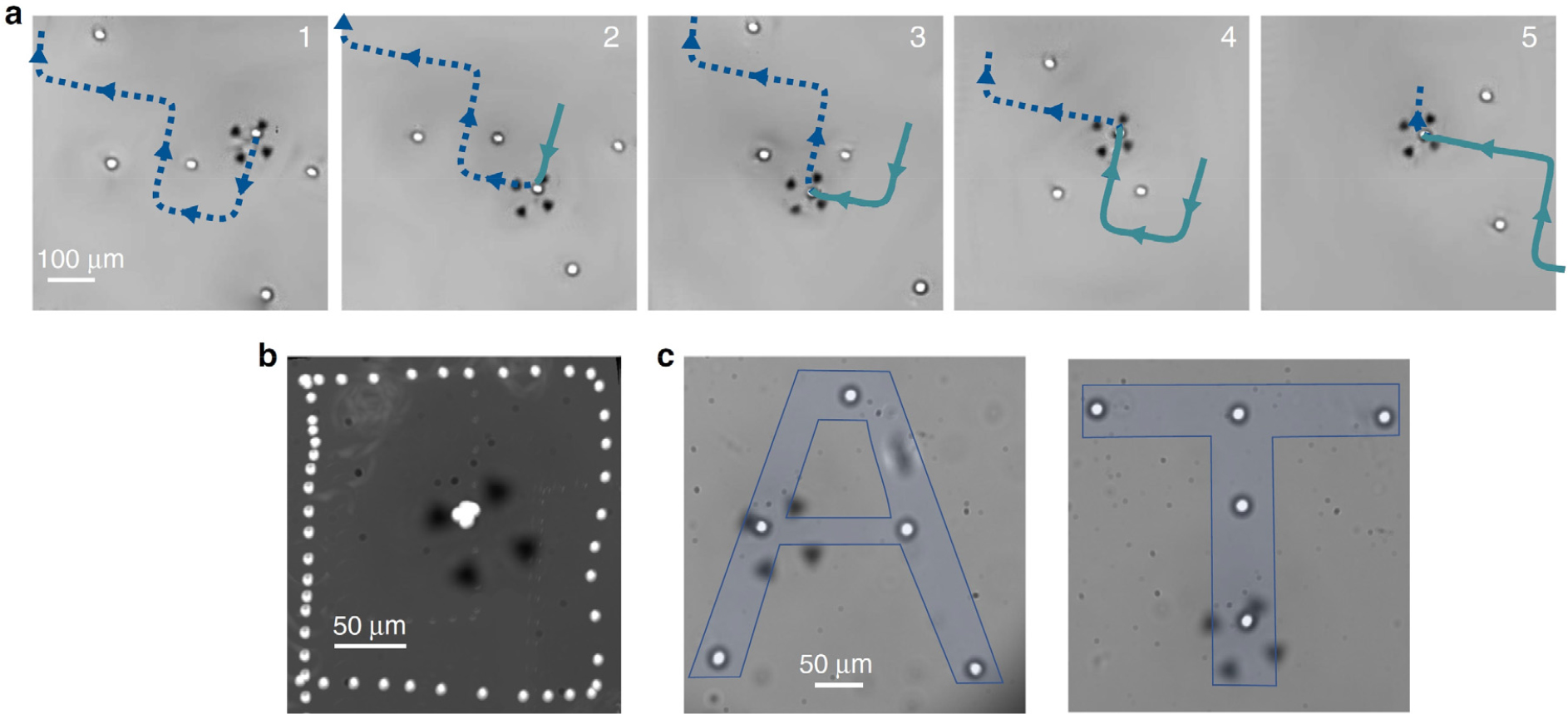
Fig. 2.
(Color available online) (a) A series of images depicting the targeted manipulation of a human breast cancer cell (MDA-MB-231) amidst neighboring cells is presented. The utilization of a blue dotted line and a green continuous line facilitates the visualization of the anticipated and preceding trajectory undertaken by the cell, respectively. (b) An illustrative image showcases the square-shaped relative motion exhibited by a trapped cell 1, positioned at the focal point of the picture, as it orbits around another cell 2. This phenomenon is achieved by overlaying the images of the two cells within the frame of reference of the trapped cell. In this particular frame of reference, the successive positions of cell 2 manifest as a geometrically precise square pattern. (c) A manipulative process involving ten MDA-MB-231 cells is captured in a visually compelling manner. The image portrays the control and arrangement of these cells to achieve a desired outcome. Fig. 2 is reprinted with permission from Springer Nature.[26]
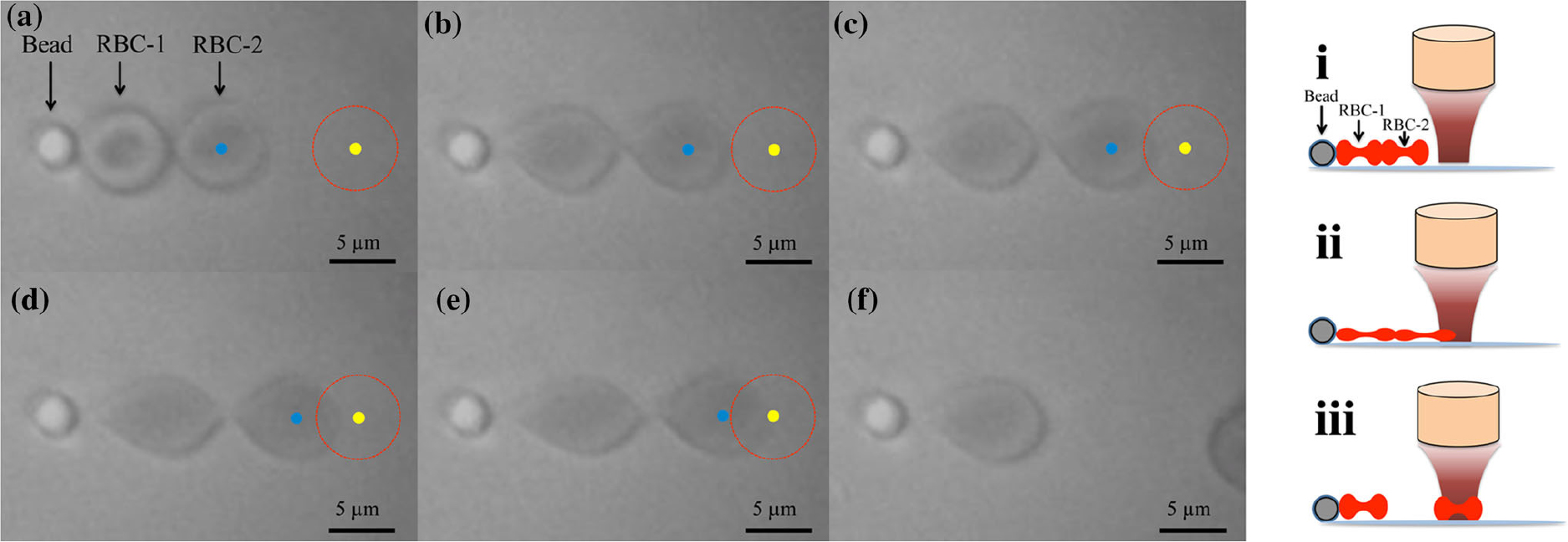
Fig. 3.
(Color available online) Experimental images and schematic diagram depicting the measurement of Red Blood Cell (RBC) forces: (a) The experimental setup involved attaching a poly-L-lysine coated microsphere to the bottom of the dish along with an RBC aggregate. A fixed transducer was positioned, and the input voltage was incrementally increased, commencing from 0 Vpp. (b-d) With the gradual increase in input voltage, the transducer exerted forces on the RBC-2, leading to its deformation and subsequent entrainment within the acoustic trap. (e) At an input voltage of 7.94 Vpp, the forces exerted by the transducer caused complete detachment of RBC-2 from RBC-1. (f) Microscopic images were captured to illustrate the disaggregation of RBCs. The red circles correspond to the acoustic trap zone, while the yellow solid circles represent the center of the acoustic trap. The blue solid circles indicate the center of RBC-2. These experimental images and schematic diagram provide insights into the process of measuring inter-RBC forces, demonstrating the successful manipulation and detachment of RBCs through acoustic trapping techniques. Fig. 3 is reprinted with permission from Springer Nature.[14]
In SBAT, the inter-RBC force is directly assessed using a pre-calibrated trapping force generated by a 410 MHz LiNbO3 focused transducer (Fig. 3). This transducer produces a focused beam narrower than the dimensions of an RBC, thereby minimizing any disturbance to neighboring cells during the trapping experiments. To calibrate the trapping force exerted on a single RBC by the 410 MHz transducer, an aspiration micropipette approach is employed. Through this method, the magnitude of inter-RBC forces was measured and found to be 391.0 pN in a specific study.[14]
Looking ahead, future endeavors will aim to utilize this technology to evaluate the interactive forces between various cell types, including adhesive forces between white blood cells and the endothelial cells lining the blood vessel surface. By expanding the application of these techniques, we can gain further insights into the intricate cellular interactions that contribute to physiological and pathological processes, advancing our understanding of complex biological systems.
3.2 Cell deformation using SBAT
Extensive research has been dedicated to investigating the mechanical properties of biomaterials, tissues, and cells due to their crucial role in tissue responses, cellular functions, and regulations. Among these, the cytoskeleton stands out as the most significant cellular mechanical component, capable of mediating cell responses by modulating the biomechanical environment through factors such as cell shape, deformation, and external pressure.
The morphological and biomechanical characteristics of cancer cells hold notable relevance not only to various blood disorders and infectious diseases but also to cancer development. Numerous studies have established a strong association between the mechanical stiffness levels of cancer cells and their invasive potential. Therefore, exploring the mechanical aspects of cells holds immense potential in predicting, evaluating, and understanding such diseases within the medical and clinical domains.
By investigating cell mechanics, researchers can gain valuable insights into disease progression, develop predictive models, and evaluate treatment efficacy. Furthermore, the exploration of cell mechanics offers opportunities for the development of novel diagnostic tools, therapeutic interventions, and personalized treatment strategies, with the ultimate aim of improving patient outcomes in the medical and clinical fields.
Acute Lymphoblastic Leukemia (ALL) cells are prevalent in childhood cancer and leukemia cases, constituting a significant contributor to cancer-related mortality, particularly among individuals below the age of 20. To gain deeper insights into drug resistance mechanisms, there has been a growing interest in exploring the mechanical properties of ALL cells as a novel characteristic. In that work, they focused on investigating the mechanical properties of four primary pre-B ALL cells following chemotherapeutic treatment (Fig. 4). Leveraging SBAT, they successfully assessed the deformability of ALL cells while suspended. The utilization of SBAT proved to be a valuable tool in evaluating the mechanical behavior of ALL cells and shedding light on their response to chemotherapeutic interventions.
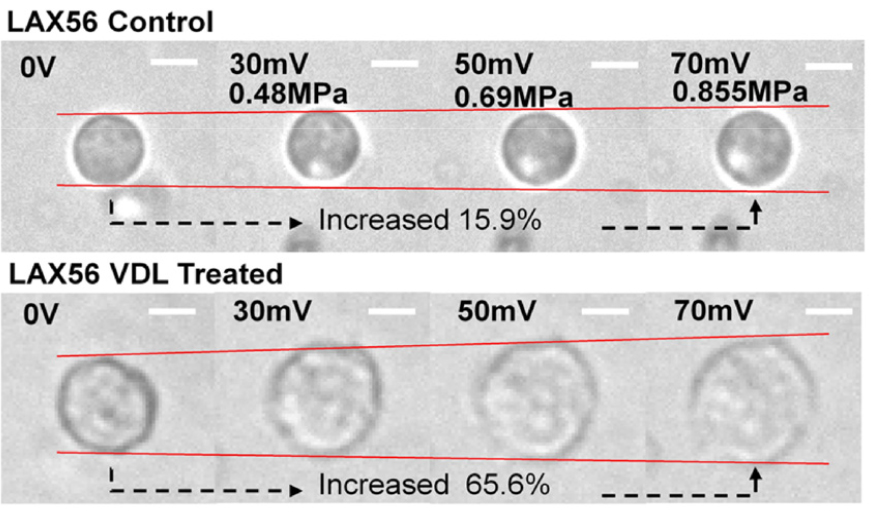
Fig. 4.
(Color available online) Cell area measurements were performed on ALL cells (LAX56) in two experimental conditions: the control group (upper row) and after 7 days of treatment with Vincristine, Dexamethasone, and L-asparaginase (VDL) (lower row). Prior to acoustic trapping, the cell areas were quantified. The white bars depicted in the images indicate a scale of 10 μm, providing a reference for size comparison. Fig. 4 is reprinted with permission from Springer Nature.[16]
In addition, the mechanical properties of breast cancer cells carry significant importance in understanding the behavior and progression of breast cancer. The mechanical properties of breast cancer cells, such as their stiffness, deformability, and adhesion, have been found to be correlated with tumor aggressiveness and the potential for metastasis. Highly invasive breast cancer cells often exhibit distinct mechanical characteristics compared to less aggressive cells. Quantifying the mechanical properties of breast cancer cells can provide insights into their ability to invade surrounding tissues, migrate, and form metastatic lesions. By understanding the mechanical behavior of breast cancer cells, researchers can potentially identify more aggressive tumor subtypes and develop strategies to inhibit metastatic spread.
Also, the mechanical properties of breast cancer cells can influence their response to various therapeutic interventions. Studies have shown that drug-resistant breast cancer cells may exhibit altered mechanical properties compared to drug-sensitive cells. By quantifying the mechanical properties, such as cell stiffness or deformability, researchers can potentially predict treatment response and identify drug-resistant breast cancer cells. This information can aid in the development of personalized treatment approaches and the identification of novel therapeutic targets to overcome drug resistance. Understanding the mechanical properties of breast cancer cells contributes to a deeper understanding of tumor behavior, provides insights into treatment response, and helps in identifying potential targets for therapeutic interventions. By investigating the mechanical characteristics of breast cancer cells, researchers aim to improve prognostication, refine treatment strategies, and ultimately improve patient outcomes in the field of breast cancer research and clinical practice.
This work presents the development of a novel diagnosis tool utilizing an in vitro acoustic tweezers platform integrated with a high-frequency (50 MHz) ultrasonic transducer (Fig. 5). The platform enables label-free trapping of human breast cancer cells, offering promising applications in the field of cancer research. To standardize the biomechanical properties of the cells, cell-mimicking phantoms and Agarose Hydrogel Spheres (AHSs) were utilized. By conducting an analytical comparison of the deformability levels between the cancer cells and the AHSs, the mechanical properties of the cells were indirectly measured by interpolating the Young’s moduli of the AHSs. By quantifying the mechanical properties of cancer cells through SBAT and utilizing standardized reference materials, such as AHSs, the paper provides a robust framework for indirectly measuring and characterizing the mechanical behavior of breast cancer cells.
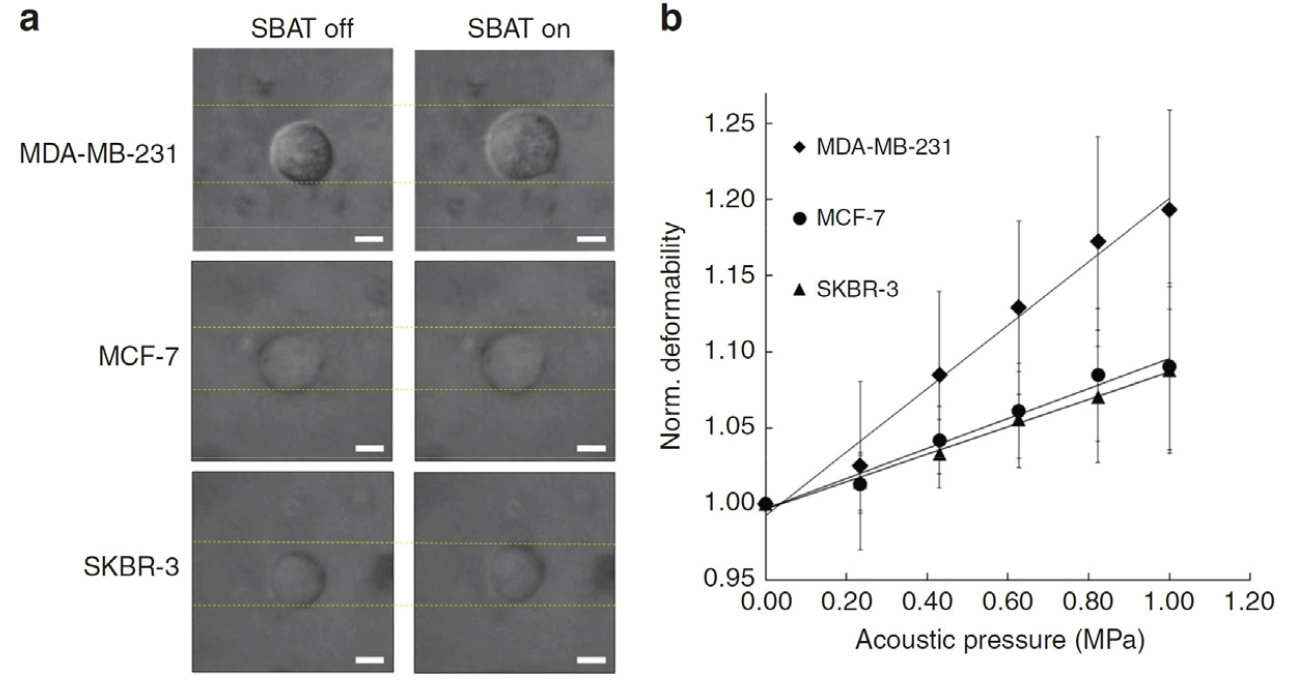
Fig. 5.
Deformability analysis of MDA-MB-231, MCF-7, and SKBR-3 cells. (a) Bright-field images depicting the area changes of MDA-MB-231, MCF-7, and SKBR-3 cells with and without the application of single-beam acoustic tweezers (SBAT). Scale bars are provided to indicate a length of 10 μm, enabling visual comparison of the cell deformability across different cell lines. (b) Normalized area changes of the trapped cells at various acoustic pressures: 0.00 MPa, 0.23 MPa, 0.43 MPa, 0.63 MPa, 0.82 MPa, and 1.00 MPa. The presented values for each cell line represent the average of 20 samples, ensuring reliable and statistically significant observations regarding the deformability characteristics of the cells under different acoustic pressure conditions. Fig. 5 is reprinted with permission from Springer Nature.[17]
IV. Conclusions
In conclusion, SBAT demonstrate remarkable versatility and hold immense potential in various fields, including biology and medicine. Their ability to separate particles and manipulate single cells makes them a promising alternative to conventional tools. Over the past few decades, SBAT has undergone significant advancements, evolving from a particle trapping method to a sophisticated platform capable of precise cell translation, separation, and deformation. These advancements have propelled SBAT to the forefront of bioparticle and cell manipulation, attracting attention from the scientific community.
This comprehensive review has explored the foundational principles of SBAT and their influences on biomedical applications such as particle/cell separation and controlled deformation of single cells. By providing a detailed understanding of the operational methodologies and fundamental principles of SBAT, this review establishes a solid conceptual framework for comprehending the advancements achieved in this field. Moving forward, further research and development in SBAT technology hold great promise for addressing challenges in diagnostics, single-cell studies, and beyond. Continued advancements in SBAT capabilities and applications will contribute to advancing our knowledge in diverse domains and ultimately pave the way for innovative solutions in biology and medicine.